How to Lock Carbon in the Soil Permanently
Large-scale carbon sequestration is a significant challenge, especially when considering competition for land and resources among different techniques. In this article we report the findings of Buss et al.[4] concerning the synergistic interactions between techniques and soil processes and how they can enhance carbon sequestration potential. The study aims to identify limitations, assess interactions, and define strategies for integrating these methods into agro-ecosystems for large-scale carbon sequestration.
Human-induced climate change is already causing severe effects on the environment, economy, and our way of life.[1] Simply reducing carbon dioxide emissions will no longer be enough to prevent further dangerous impacts. There is now a need to capture and store carbon in order to reduce the quantity of greenhouse gasses in the atmosphere. One approach is through nature-based solutions or geotherapy, enhancing natural processes to sequester carbon, which offers an alternative to proposed industrial carbon capture and storage using geoengineering tactics.[2,3]
Nature-based methods have positive environmental effects and can potentially be economically beneficial due to their ability to increase natural resources and agricultural productivity. Examples of nature-based solutions include agroforestry, soil organic carbon (SOC) sequestration, biochar production, and enhanced rock weathering (Figure 1).
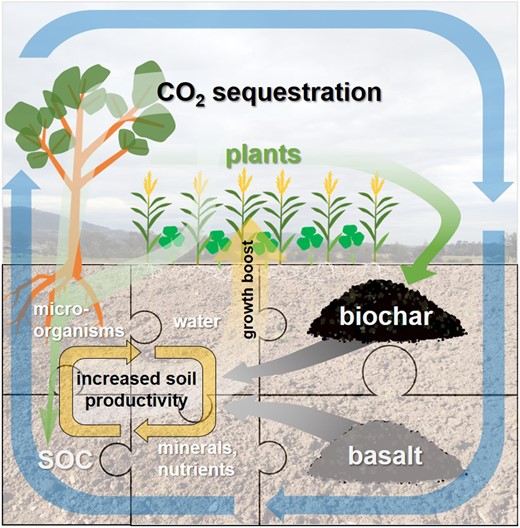
Geotherapy
Plants convert CO2 into sugars, which are distributed in the plant and soil. Increased plant biomass leads to higher soil organic carbon. Undisturbed plant carbon remains stable for over 100 years. Practices like cover cropping and agroforestry can boost carbon stocks. Agroforestry, in particular, can significantly increase biomass and soil organic carbon.
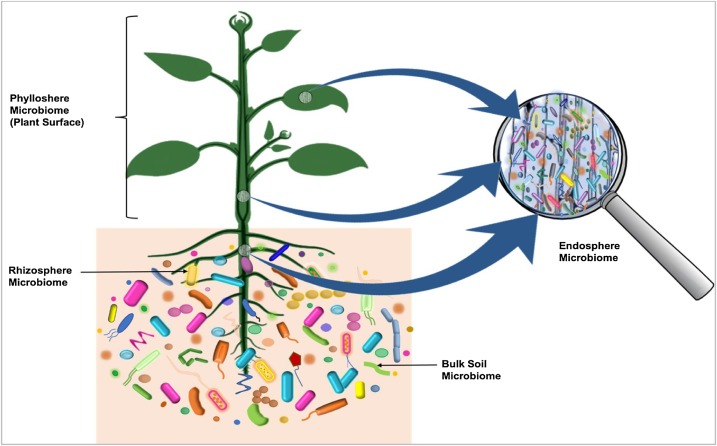
Microorganisms play a key role in converting plant carbon into stable soil organic carbon. Increasing the amount of carbon stored in soils requires protecting it in soil aggregates and binding it to minerals. However, these levels can saturate and can be disturbed by factors like overgrazing and land-use change. Nutrient and water limitations can restrict global plant biomass accumulation. Disturbances like fires, land-use changes, and climate change can release stored carbon dioxide. Accounting for these variables, carbon sequestration efficiency is a measure of how effectively plant carbon is transformed into stable form, safely trapped in the soil. Agricultural practices can be implemented to increase carbon sequestration at low or no cost.[5]
For example, biochar is a stable form of carbon produced by heating biomass without oxygen. It has a long lifespan and can offset carbon emissions over time. However, its use is limited by biomass availability and processing costs.[6]
Another method is enhanced weathering, which involves crushing silicate rocks and applying them to soil to accelerate the process of rock dissolution. After application, the dust slowly dissolves in leaching water, releasing important nutrients for the plants such as manganese, calcium, phosphate, potassium and others into the soil. During weathering, carbon dioxide is captured and stored in the form of dissolved bicarbonate. Basalt rocks are commonly used due to their low toxicity and their broad-spectrum content of elements and trace minerals that promote plant growth. The efficacy of basalt weathering depends on factors such as particle size, climate, soil conditions, and biological activity. However, the actual rates and carbon drawdown potential of basalt weathering remain uncertain.[7]
Enhanced carbon capture from geotherapy
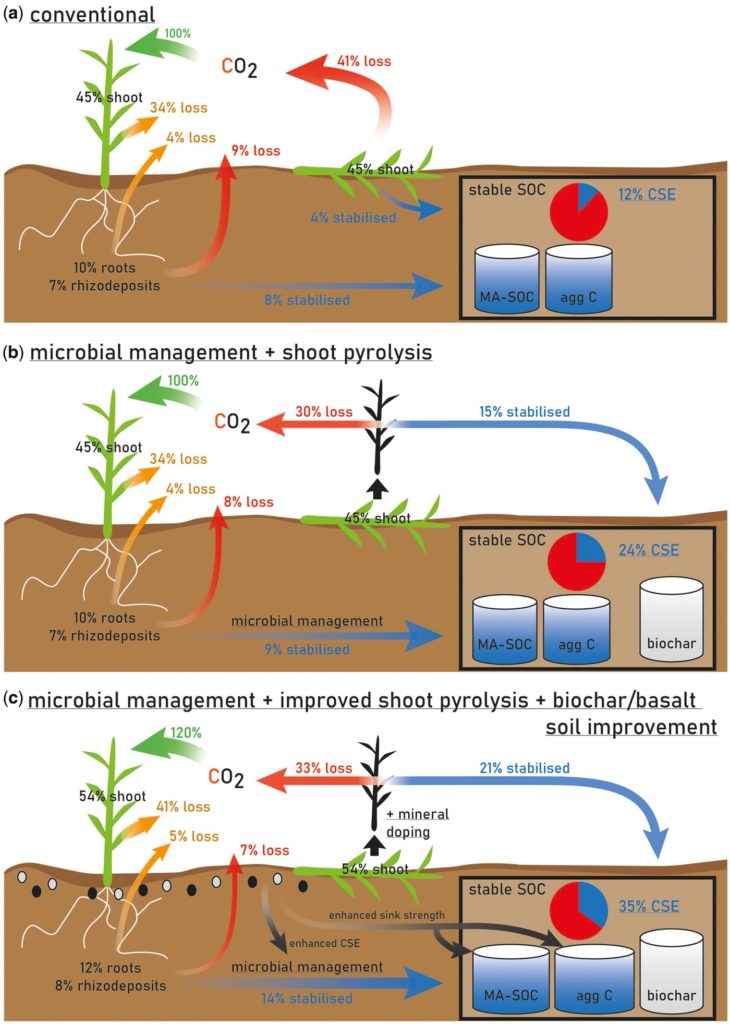
Figure 2 compares the potential of carbon sinks in the conventional way (Figure 2a), with shoot pyrolysis/biochar application (Figure 2b) and with biochar/rock dust applications with soil improvement (Figure 2c). The green arrows represent photosynthesis, orange represents autotrophic respiration (plant cells that don’t produce their energy through photosynthesis but through respiration), red represents heterotrophic respiration (microbial respiration). Percentages are examples of values found in the literature and are presented for illustrative purposes. They vary according to the system under investigation (soil, plant type, and so on).
In conventionally managed plots, carbon dioxide is captured through photosynthesis, which stores carbon in the plant structure to be used as an energy source through plant respiration. Microorganisms degrade plant carbon through respiration but also foster its conversion into stabilized forms of mineral associated soil organic carbon (labeled ‘MA SOC’ in Figure 2); aggregate carbon (labeled ‘agg C’ in figure 2) from roots decomposition residues, roots exudates and shoot decomposition residues. The quantity of aboveground plant carbon (mainly shoots) that ends up into a stabilized form is lower than for belowground plant carbon (roots and rhizodeposits.)
Using shoot pyrolysis and biochar application, the amount of stable carbon stemming from the shoot is dramatically increased. Pyrolysis directly transforms atmospheric carbon captured by plants into a form that is significantly more stable than unpyrolyzed biomass, increasing the time carbon remains in soils.
Using a combination of biochar and rock dust application, the amount of carbon dioxide absorbed by plants compared to the conventional way is much higher due to the improved properties of the soil (helped by slow nutrient release). Altogether, this results in enhanced carbon sequestration via boosting of the existing cycle. As mentioned above, rock weathering also captures carbon dioxide in the form of carbonate, which further increases the already enhanced carbon sequestration efficiency.
How carbon capture techniques help each other
Figure 3 represents how nutrients/water leaching and atmospheric carbon flow behave in agricultural ecosystems. In standard agricultural systems, the leaching of nutrients and water causes significant economical and environmental issues (Figure 3a.) Soils play a crucial role in retaining water and nutrients, but its poor management can lead to significant losses.
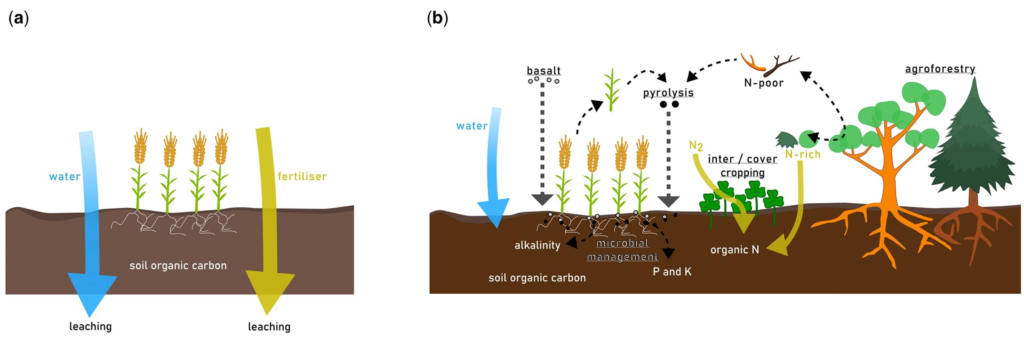
Soil organic carbon has a high cation exchange capacity (CEC), the ability to retain positively charged nutrients thanks to negative surface charges. Furthermore, the application of rock dust and biochar can enhance the CEC in soils by providing further negatively charged surfaces. A large-scale analysis demonstrated that 50% less nitrogen fertilizer was needed when the soil organic carbon content in soils was increased from 0.5% to 1%.
The ideal system would be able to capture precipitation rapidly and retain it for a long time. Soil texture and the particle size distribution is a key factor when considering how water acts within soil. In clay-rich soils, the fine packing of particles restricts water infiltration and movement within the soil but helps retain water. In contrast, sandy soils and their coarse grain arrangement facilitate water infiltration but have drastically decreased retention.
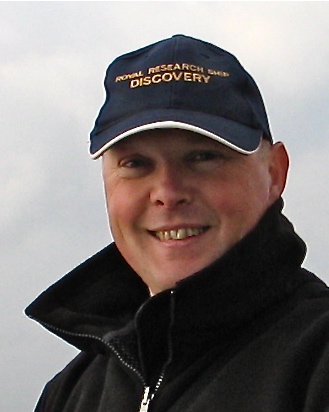
Soil structure also plays a crucial role in soil hydraulic functions. Increased soil carbon content, root biomass and the abundance of organisms have been correlated with high soil aggregation. Therefore, accumulation of soil organic carbon and rock dust application can help water infiltration and retention. Biochar is also likely to change soil texture, thanks to its particle size, as well as adding more components that can improve soil structure.
Studies have shown that nitrogen increases soil organic carbon formation and stability. During biomass pyrolysis, most of the nitrogen is lost into an unavailable form for soils. Therefore, it is interesting to make a difference between nitrogen-rich green material and nitrogen-poor green materials. The first one should not be considered for biochar production as it can be used to increase the amount of captured carbon in the form of compost, meanwhile the latter has less nitrogen to provide and can be pyrolyzed with no regrets.
Biogeochemical management of soils through strategic application of biochar and rock dust has the potential to spark beneficial cycles that increase water use efficiency, plant growth and soil organic carbon accumulation. The stabilization of carbon in the soils removes carbon dioxide from the atmosphere, thus helping to fight climate change. Rendering nutrients readily available for plants to uptake, while mitigating leaching, would help with the growth of healthy crops and forests. Better water management with the prevention of leaching can also be attained which in turn helps plants in drylands. These considerations for forestry and cropland management have the potential to reverse runaway global warming and trigger better outcomes for humankind.
Nicolas Bellomo has just completed his PhD in material science and engineering (chemistry). He is passionate about sustainability, his main motivation for his career path and joining RTE as a science writer. He also graduated with a bachelor and master’s degree in bioengineering. His last name sounds Italian but he is actually located in Luxembourg and Belgium. He likes traveling and meeting new cultures which is why he has done his share of travels (Iceland, USA, New Zealand, Vietnam and many more).
Works cited
[1] Hansen, J., Sato, M., Kharecha, P., von Schuckmann, K., Beerling, D. J., Cao, J., Marcott, S., Masson-Delmotte, V., Prather, M. J., Rohling, E. J., Shakun, J., Smith, P., Lacis, A., Russell, G., and Ruedy, R. (2017) Young people’s burden: requirement of negative CO2 emissions, Earth Syst. Dynam., 8, 577–616, https://doi.org/10.5194/esd-8-577-2017.
[2] M. Bui, C. S. Adjiman, A. Bardow, E. J. Anthony, A. Boston, S. Brown, P. S. Fennell, S. Fuss, A. Galindo, L. A. Hackett, J. P. Hallett, H. J. Herzog, G. Jackson, J. Kemper, S. Krevor, G. C. Maitland, M. Matuszewski, I. S. Metcalfe, C. Petit, G. Puxty, J. Reimer, D. M. Reiner, E. S. Rubin, S. A. Scott, N. Shah, B. Smit, J. P. M. Trusler, P. Webley, J. Wilcox, N. Mac Dowell (2018). Carbon capture and storage (CCS): the way forward. Energy & Environmental Science, 11(5), 1062-1176.
[3] D’Alessandro, D. M., Smit, B., & Long, J. R. (2010). Carbon dioxide capture: prospects for new materials. Angewandte Chemie International Edition, 49(35), 6058-6082.
[4] Buss, W., Yeates, K., Rohling, E. J., & Borevitz, J. (2021). Enhancing natural cycles in agro-ecosystems to boost plant carbon capture and soil storage. Oxford Open Climate Change, 1(1), kgab006.
[5] Pausch, J., & Kuzyakov, Y. (2018). Carbon input by roots into the soil: quantification of rhizodeposition from root to ecosystem scale. Global change biology, 24(1), 1-12.
[6] O’Laughlin, J., & McElligott, K. (2009). Biochar for environmental management: science and technology, in Johannes Lehmann, Stephen M. Joseph (Eds.), Earthscan, London UK (2009), 448, 535-536.
[7] D. J. Beerling, J. R. Leake, S. P. Long, J. D. Scholes, J. Ton, P. N. Nelson, M. Bird, E. Kantzas, L. L. Taylor, B. Sarkar, M. Kelland, E. DeLucia, I. Kantola, C. Müller, G. Rau, J. Hansen (2018). Farming with crops and rocks to address global climate, food and soil security. Nature plants, 4(3), 138-147.
Support us on Patreon
Thank you for joining us today! Please become a member of RTE and support us on Patreon. Unlike many larger organizations, we work with a team of determined and passionate volunteers to get our message out. We aim to continue to increase the awareness of remineralization to initiate projects across the globe that remineralize soils, grow nutrient dense food, regenerate our forests’ and stabilize the climate – with your help! If you can, please support us on a monthly basis from just $2, rest assured that you are making a big impact every single month in support of our mission. Thank you!
Got something to say?